Inspiring Plenary Lectures by International Experts
Plenary speaker: Ifan Stephens | Imperial college London
Ifan Stephens is a Professor of Electrochemistry at Imperial College London. His research focuses on enabling large-scale electrochemical energy conversion for fuels and chemicals, key to the renewable energy transition.
He has published extensively on oxygen evolution, nitrogen reduction, CO₂ reduction, and battery degradation. His awards include the MIT Peabody Visiting Associate Professorship (2015), ERC Consolidator Grant (2021), RSC John Jeyes Award (2021), Clarivate Highly Cited Researcher (2022–present), and RSC Geoffrey Barker Medal (2024). His work on H₂O₂ electrosynthesis led to the HPNow spinout, raising €30M and commercialising electrolysers in 20 countries.
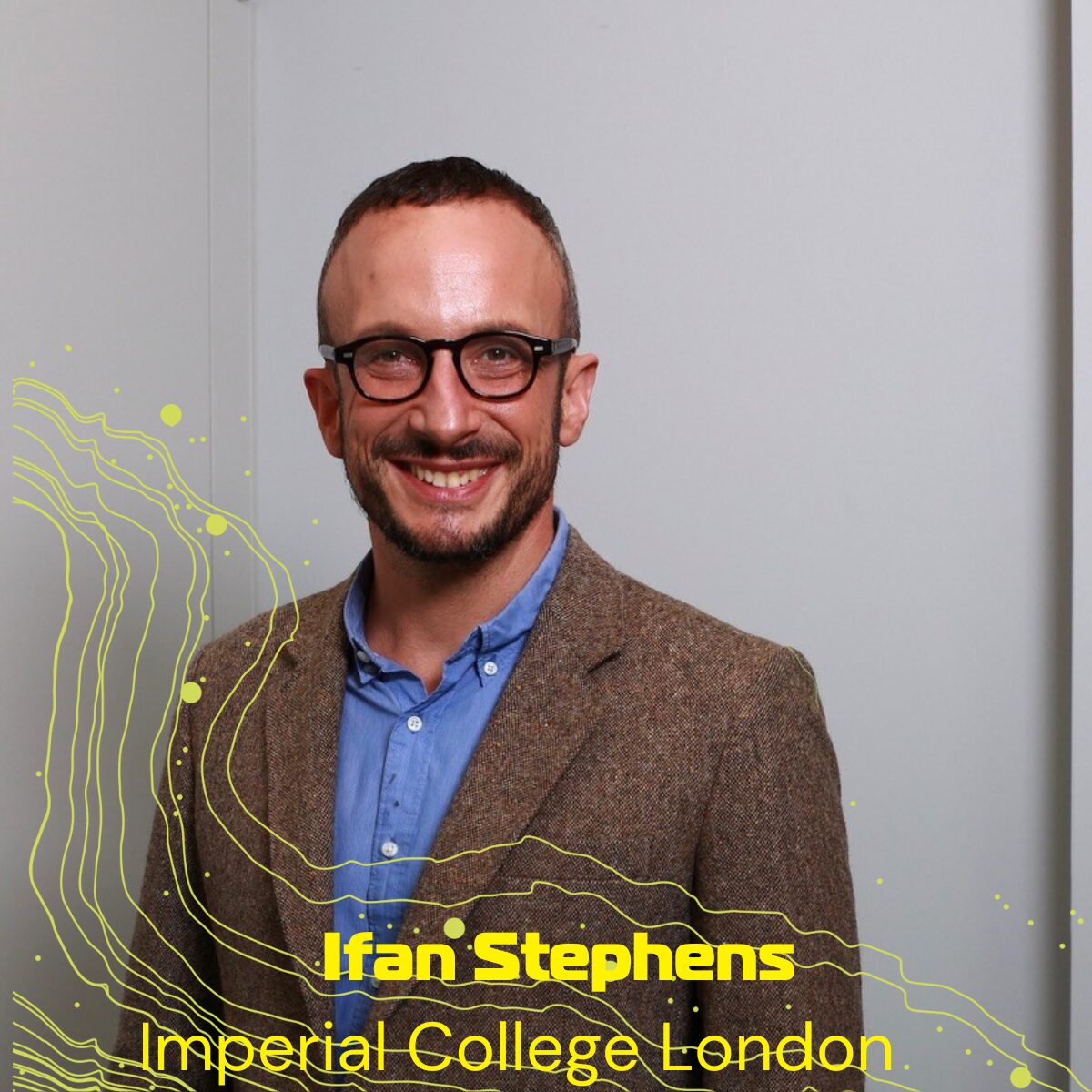
Title: Electrochemical Challenges Across Green Hydrogen, Ammonia, and Battery Technologies
Electrochemistry is central to achieving a net-zero society. While fuel cells and batteries are crucial for decarbonising transport, electrolysers offer a sustainable route to producing fuels and chemicals, such as hydrogen and ammonia. Intriguingly, many electrochemical reactions we aim to accelerate in electrosynthesis—such as hydrogen evolution in water electrolysis—are exactly those we strive to suppress in batteries and nitrogen reduction. This talk will explore how insights from electrocatalysis inform battery science and vice versa.
I will present mechanistic studies on (i) oxygen evolution in iridium-based catalysts for water electrolysis, (ii) nitrogen reduction to ammonia in organic electrolytes, and (iii) parasitic gas evolution in lithium-ion batteries. These studies integrate electrochemical mass spectrometry, operando optical spectroscopy, cryo-electron microscopy, secondary ion mass spectrometry, X-ray photoelectron spectroscopy, and density functional theory. By combining these techniques, we develop a holistic understanding of the factors governing these technologically critical reactions.
Plenary speaker: Marnix Wagemaker | Technical University of Delft
Prof.dr.ir. M. Wagemaker received his PhD in physics at Delft University Technology (2003) and is full professor in electrochemical energy storage, heading the section Storage of Electrochemical Energy since 2017 at the Delft University of Technology in the Netherlands. Currently he holds a VICI grant on battery research, coordinates the national BatteryNL research consortium (NWA-ORC national project) and is coordinating the TUD e4-battery platform focussing on the development of future circular battery technology. His interests include fundamental battery processes, studied by simulation/modelling (atomic scale DFT and meso-scale modelling) and characterization (Neutron and X-ray scattering, solid state NMR, Electrochemistry) thereof, aiming at improved understanding and the development of next generation battery materials.
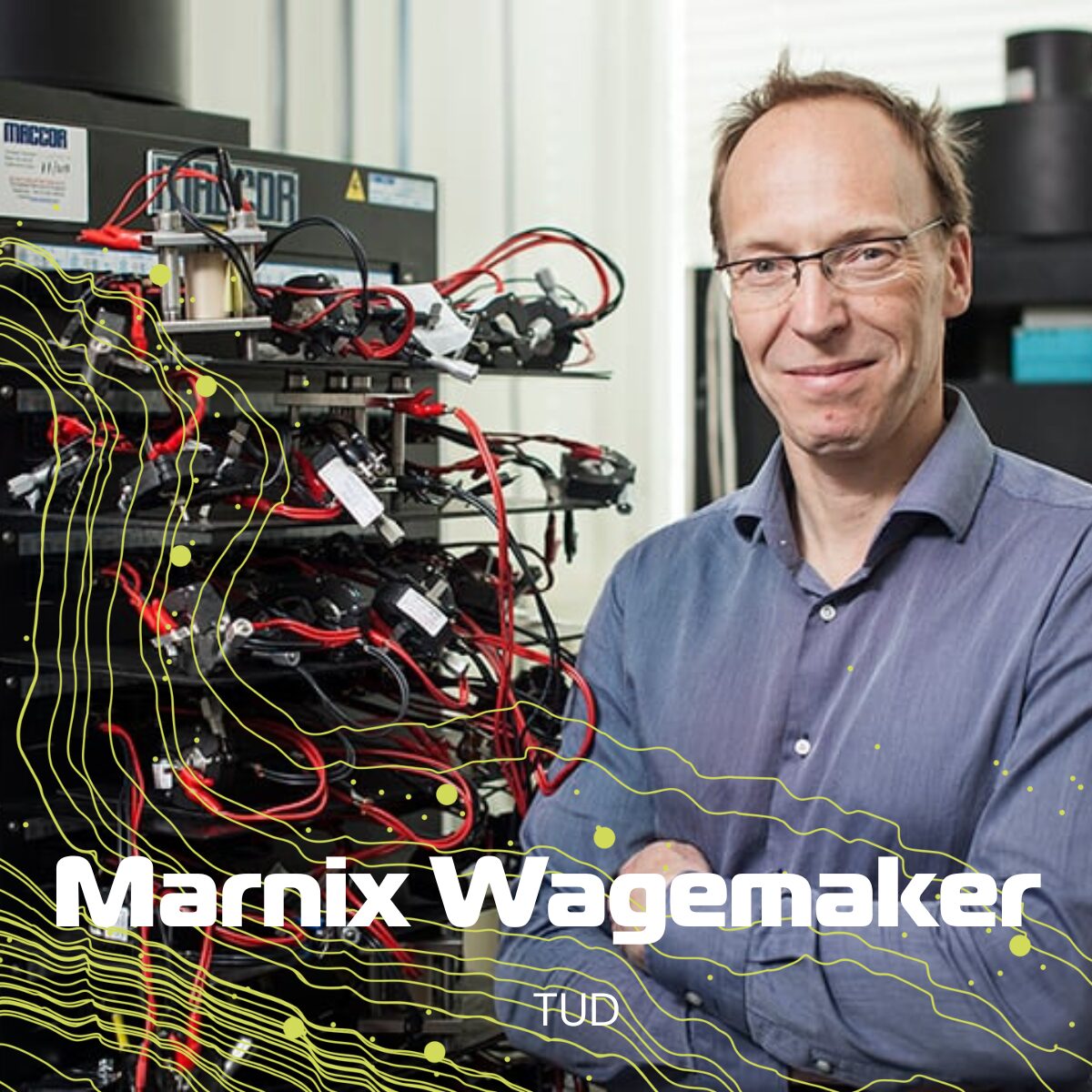
Abstract
Battery technology has enabled large scale mobility, mobile equipment and stabilizing the grid on short timescales. Yet there is much to wish for, larger energy densities are required to extend driving range for instance, improved safety is paramount with the scale that batteries are being introduced i0n our daily lives, use of abundant and less critical raw materials, and as well as more sustainable processes are demanded. More battery chemistries enter the market and countless battery chemistries are being explored addressing one or more of these wishes. All these current and future battery generations/chemistries have one common challenge, reversibility. Extremely high reversibility is demanded to reach thousands of charge-discharge cycles, a prerequisite to arrive at acceptable battery cost and environmental impact. In this talk we look at what processes challenge the reversibility in some of the next generation high energy density battery chemistries, specifically focussing on the atomic scale design and development of electrolytes to improve this.
Speakers morning break-out sessions
Session 1: Water electrolysis - low temperature
Rik Mom – Universiteit Leiden
Rik Mom’s research is focused on finding the fundamental ingredients that make electrocatalysts stable and active. To this end, he uses operando X-ray spectroscopies to unravel the chemistry of the electrode surface, electrolyte ions and water molecules at the electrode-electrolyte interface.
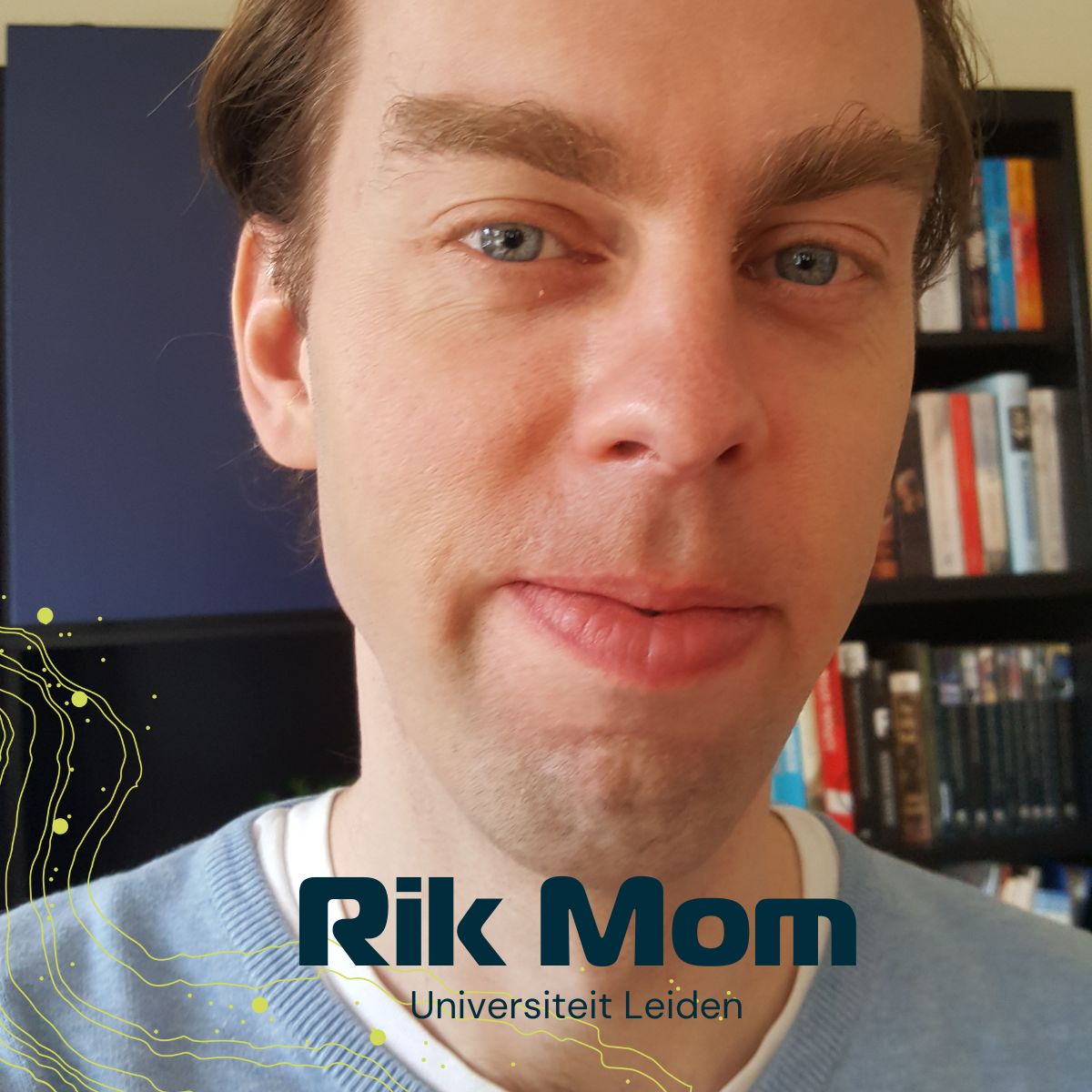
Abstract: A live view on electrolysis using in situ XPS and XAS
The interplay between the electrode surface, water, and ions at the electrode-electrolyte interface is a key factor in the performance of electrolyzers. Here, I will discuss our efforts to understand this interfacial chemistry using X-ray spectroscopy. To this end, we have designed operando X-ray photoelectron spectroscopy and (soft) X-ray absorption spectroscopy methodology that can specifically detect the individual chemistries of interfacial water, near-surface ions, and the electrode surface. Here, I will discuss case studies from both alkaline and acidic electrolysis, in which we investigated electrolyte effects and electrode restructuring.
Session 1: Water electrolysis - low temperature
Corina Andronescu earned her Ph.D. in chemical engineering from the University Politehnica of Bucharest, Romania, in 2014. In 2016, she moved to the Ruhr University Bochum as a postdoctoral researcher, and in December 2018, she was appointed as a Junior Professor at the University of Duisburg-Essen (UDE). Since March 2024, she has been a Professor of Electrochemical Catalysis at UDE. Her research focuses on discovering new electrocatalyst materials, establishing activity-structure correlations in catalysts, and understanding critical factors for integrating catalyst materials into devices.
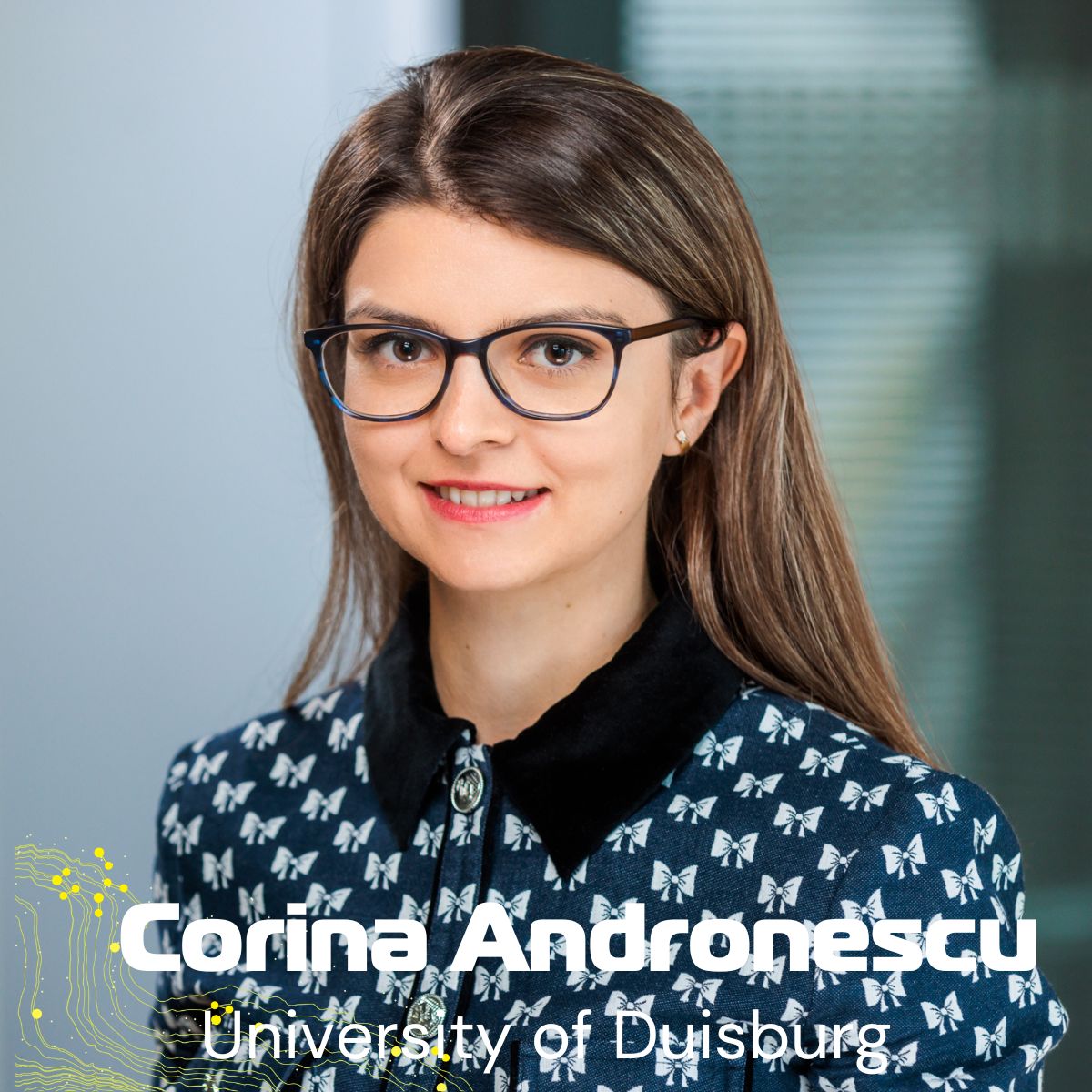
Water splitting electrocatalysts: what can we learn at the nanoscale and in device
1Chemical Technology III, Faculty of Chemistry and CENIDE; University of Duisburg-Essen, Carl-Benz-Straße199, 47057 Duisburg/Germany
Catalyst materials play an important role in enabling sustainable hydrogen production via water electrolysis. Catalyst materials can be complex in structure, often having various crystal orientations. Their effectiveness is usually tested using catalyst films, which give an average performance across all structural features. Therefore, it’s important to understand how structure relates to activity at the catalyst level to design better electrocatalyst materials. At the device level, complexity increases because the catalyst and other parts like membranes, ionomers, and operation modes can affect stability and long-term performance.
This talk will show examples of how scanning electrochemical cell microscopy (SECCM) enables the evaluation of oxygen evolution reactions (OER) catalysts at the nanoscale. I will point out key factors to be considered when testing OER catalysts with SECCM, focusing on how the atmosphere used during measurements affects the recorded activity. In the second part, I will discuss how the composition of the electrolyte and the operation mode influence the stability of low-temperature anion-exchange membrane electrolysis.
Acknowledgment: The financial support was provided by the German Research Foundation (Deutsche Forschungsgemeinschat, DFG) – CRC247 [388390466] and BMBF – project PrometH2eus (03HY105F).
Session 2: (Bio) CO2 electrochemical conversions 1
Dr. Ruud Kortlever obtained his PhD under the supervision of Prof. dr. Marc T.M. Koper in 2015 from Leiden University, on the topic of selective and efficient nanoparticulate catalysts for the electrochemical CO2 reduction reaction. After obtaining his PhD, he worked as a postdoctoral researcher at the Joint Center of Artificial Photosynthesis (JCAP) at Caltech, in the groups of Prof. dr. Theodor Agapie and Prof. dr. Jonas C. Peters. In 2018 he moved back to the Netherlands to start his group on ‘Electrocatalysis for Energy Storage and Conversion’ (www.kortlevergroup.com) at the Department of Process & Energy of TU Delft, where he currently is an associate professor. The work of the Kortlever group focusses on electrocatalytic processes to make products that can be used as fuels or are bulk chemicals relevant for the chemical industry. The main reactions currently studied are electrochemical water splitting, CO2 and N2 electrolysis.
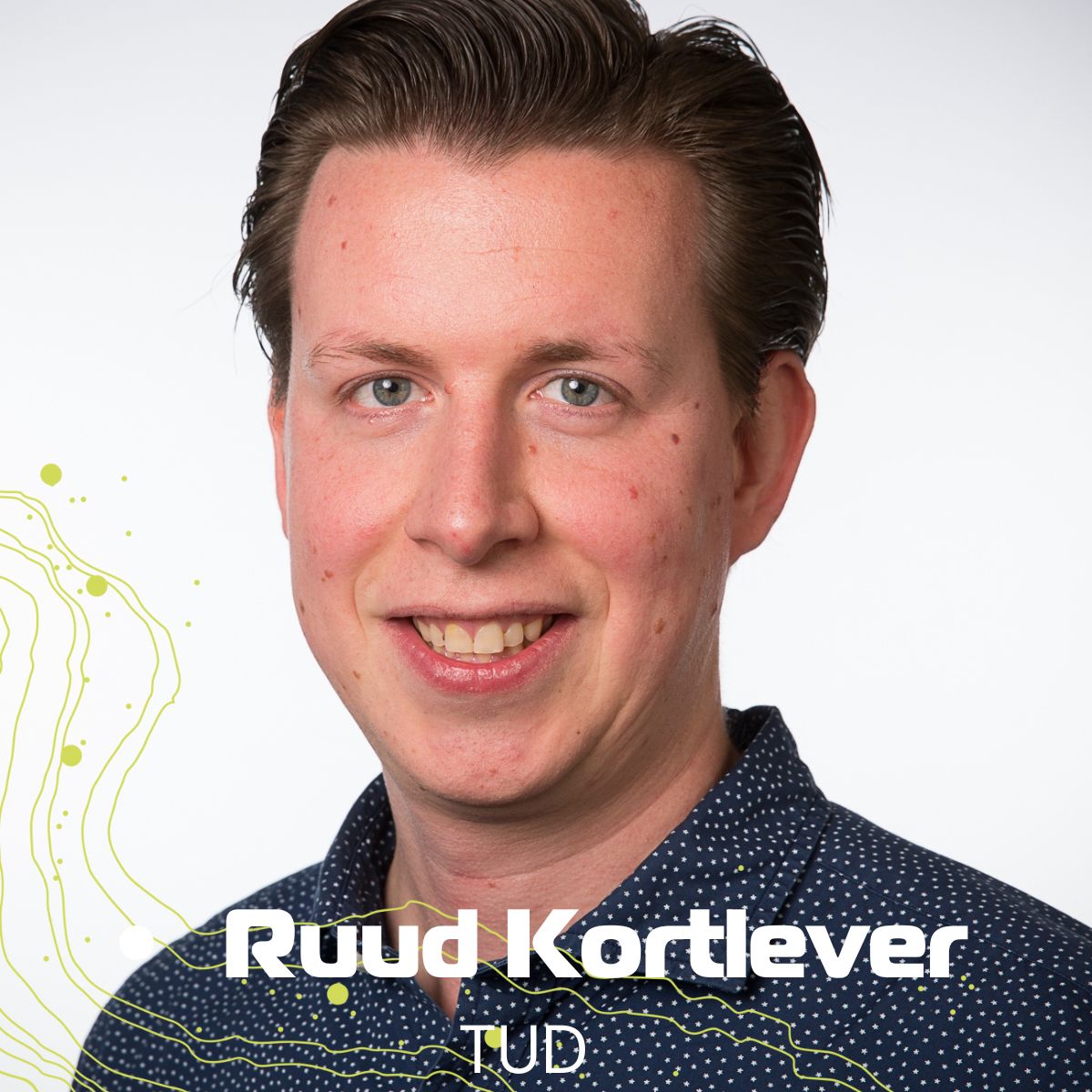
Challenges of using impure feeds for CO2 electrolysis
CO2 electrolysis to fuels or commodity chemicals, driven by renewable energy, provides a unique opportunity to both utilize CO2 and store renewable energy in chemical bonds [1]. The CO2 feedstock for the CO2 electrolysis can be obtained from various sources, such as from direct air capture (DAC) or from point sources such as the chemical industry or power plants. One of the major drawbacks of using industrial CO2 point sources is the presence of contaminants such as SO2, H2S, COS, NOx and other volatile organic compounds that could be highly detrimental to the catalysts that drive the conversion of CO2 to products.
In this talk, I will discuss the influence of various concentrations of sulphur-based gaseous impurities, specifically SO2, H2S and COS, on the selectivity, product distribution and catalyst stability during CO2 electrolysis. First, I will show the effect of SO2 impurities on the electrocatalytic performance of a N-doped carbon catalyst for CO production. Overall, this study demonstrates the strong tolerance and robustness of N-doped carbon catalysts compared to silver for the reduction of CO2 to CO in the presence of SO2 impurities. Finally, I will show that SO2, H2S and COS contaminants significantly suppress the formation of hydrocarbon products during the CO2RR on copper-based electrodes, both at low currents in a H-cell setup and at higher currents in a GDE flow cell. I will show that the suppression is related to the type of contaminant, its concentration, the cathode potential and the cell configuration. Moreover, I will provide insights on the degradation pathways and highlight the importance of these results for the design of large-scale processes that convert CO2 feeds with these impurities.
Session 2: (Bio) CO2 electrochemical conversions 1
Mariana Monteiro – Max-Planck institute
Mariana obtained her PhD in Electrochemistry (cum laude) from Leiden University in 2022. Her thesis work on electrolyte effects on CO2 reduction and H2 evolution was awarded the “DCS Thesis Award” and the “Amcel PhD Award” in 2023. After a brief Postdoc at the Interface Science Department at the Fritz Haber Institute, in Berlin, she was awarded a Minerva Fast-Track position by the Max Planck Society to establish her research group “Electrode-Electrolyte Interfaces”. Her group studies the fundamentals of surface-electrolyte interactions using classical electrochemistry and operando techniques as Surface X-Ray Diffraction.
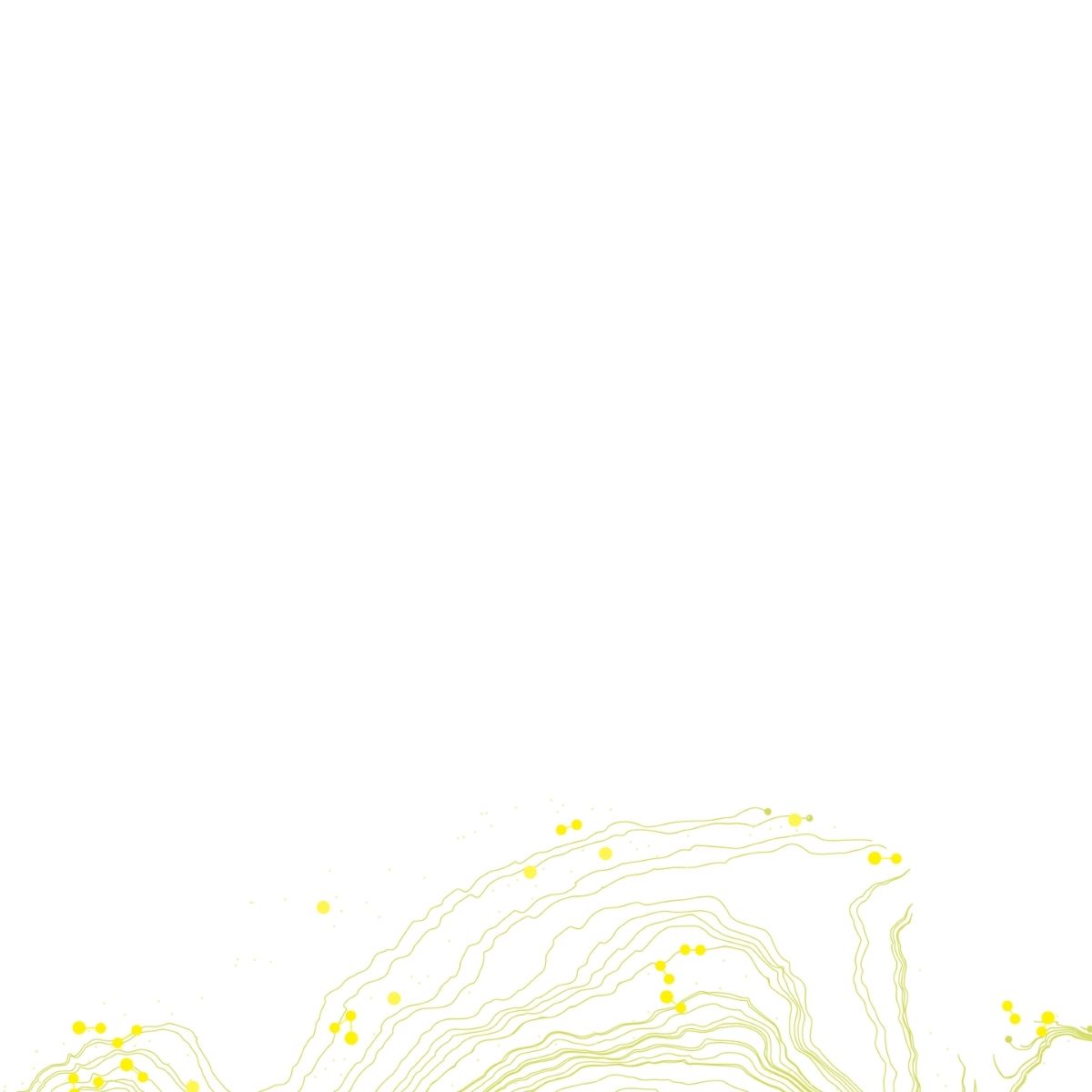
Electrolyte design for acidic CO2 electrolysis
Mariana Monteiro
Interface Science Department, Fritz Haber Institute of the Max Planck Society, Faradayweg 4-6, 14195 Berlin, Germany
Acidic electrochemical CO2 reduction offers a promising method to convert CO2 into valuable chemicals and fuels, providing advantages over neutral or alkaline systems, such as lower energy consumption and higher carbon utilization. Despite these benefits, the optimal electrolyte for acidic media remains unclear. Key challenges include ensuring catalyst stability and optimizing operating conditions, like current density and pH, to maximize efficiency and product yield. In this study, we explore CO2 reduction on copper in seven different acidic electrolytes. We investigate the impact of supporting anions and their solution chemistry on reaction selectivity across current densities of 10–160 mA/cm². The catalyst’s chemical state and evolution are monitored using operando X-ray Absorption Spectroscopy (XAS), while morphological changes are analyzed via Scanning Electron Microscopy (SEM). Our results show that selectivity correlates with the pKa and diffusion coefficient of the acids, affecting local pH and determining optimal operating currents for each electrolyte. In contrast, morphological and chemical state changes seem to play a less significant role. Finally, we provide guidelines for selecting electrolytes in low-pH CO2 electrolysis, which is crucial for advancing acidic CO2 reduction technologies.
Session 3: Electrochemical energy storage
Adriana Creatore is plasma chemist and material scientist, with a MSc and a PhD in Chemistry from the University of Bari. Since 2019, she holds a full professorship on “Material and interface design and engineering for next-generation energy technologies” at the department of Applied Physics and Science Education of TU/e. She exploits plasma chemistry, material science and thin film deposition technologies, such as atomic layer deposition (ALD), to engineer and investigate materials and interfaces (at nm level) for energy devices, ranging from metal halide perovskite-based photovoltaics to high-energy density, safe, and economically viable lithium -ion batteries.
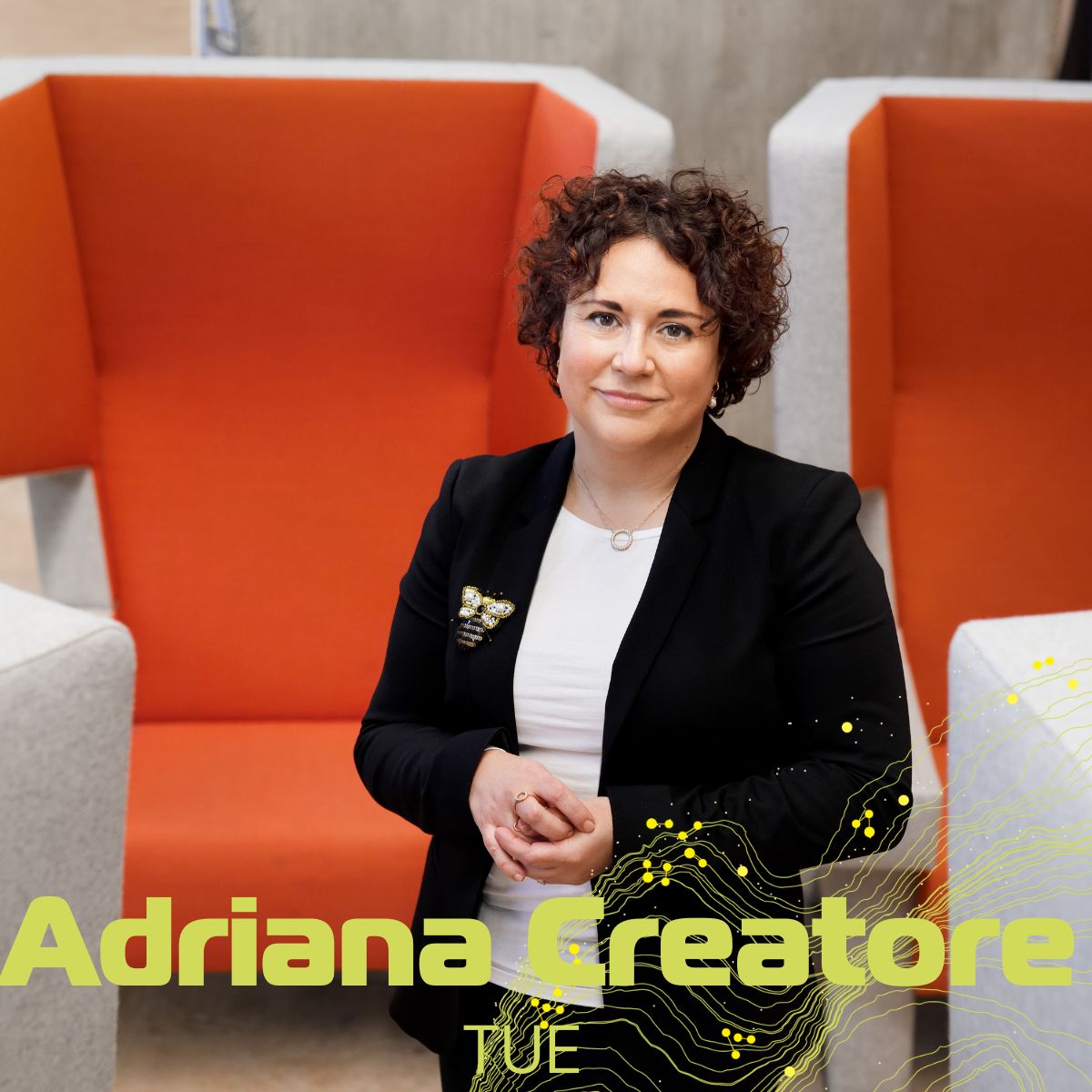
Title: Interface engineering by atomic layer deposition for next-generation Li-ion batteries
In this contribution I will discuss the state-of-the art and challenges yet to be met in material and interface science for the next-generation EV batteries. Next to the challenge of sustainability in material supply chain, manufacturing line and recycling prospects, the development of next-generation Li-ion batteries (LiBs) requires understanding and control over interface reactions that drive both the operation and degradation of the battery. To this purpose, I will introduce and reflect on the merits and opportunities offered by gas phase deposition technologies, such as atomic layer deposition (ALD), towards electrochemically and mechanically stable electrode/electrolyte interfaces. ALD delivers uniform and conformal thin films with ultimate control over thickness and chemical composition, with major opportunities for processing also on complex, 3D electrode structures. This contribution will encompass several examples of interface engineering by ALD, ranging from LiF to Li2CO3 and N-doped Li3PO4, while providing thoughts on them challenges towards implementation of ALD in a battery manufacturing line, in terms of added (manufacturing) costs vs. added value (e.g., impact on KPIs).
Session 3: Electrochemical energy storage
Evan Wenbo Zhao – Radboud University
Evan is an assistant professor at the Magnetic Resonance Research Center, Radboud University Nijmegen. His research is on developing and applying operando magnetic resonance to understand electrochemical energy storage and conversion, including redox flow battery, electrochemical ammonia synthesis, carbon dioxide reduction and lignin oxidation. Evan did his postdoc at the University of Cambridge and obtained his PhD from the University of Florida.
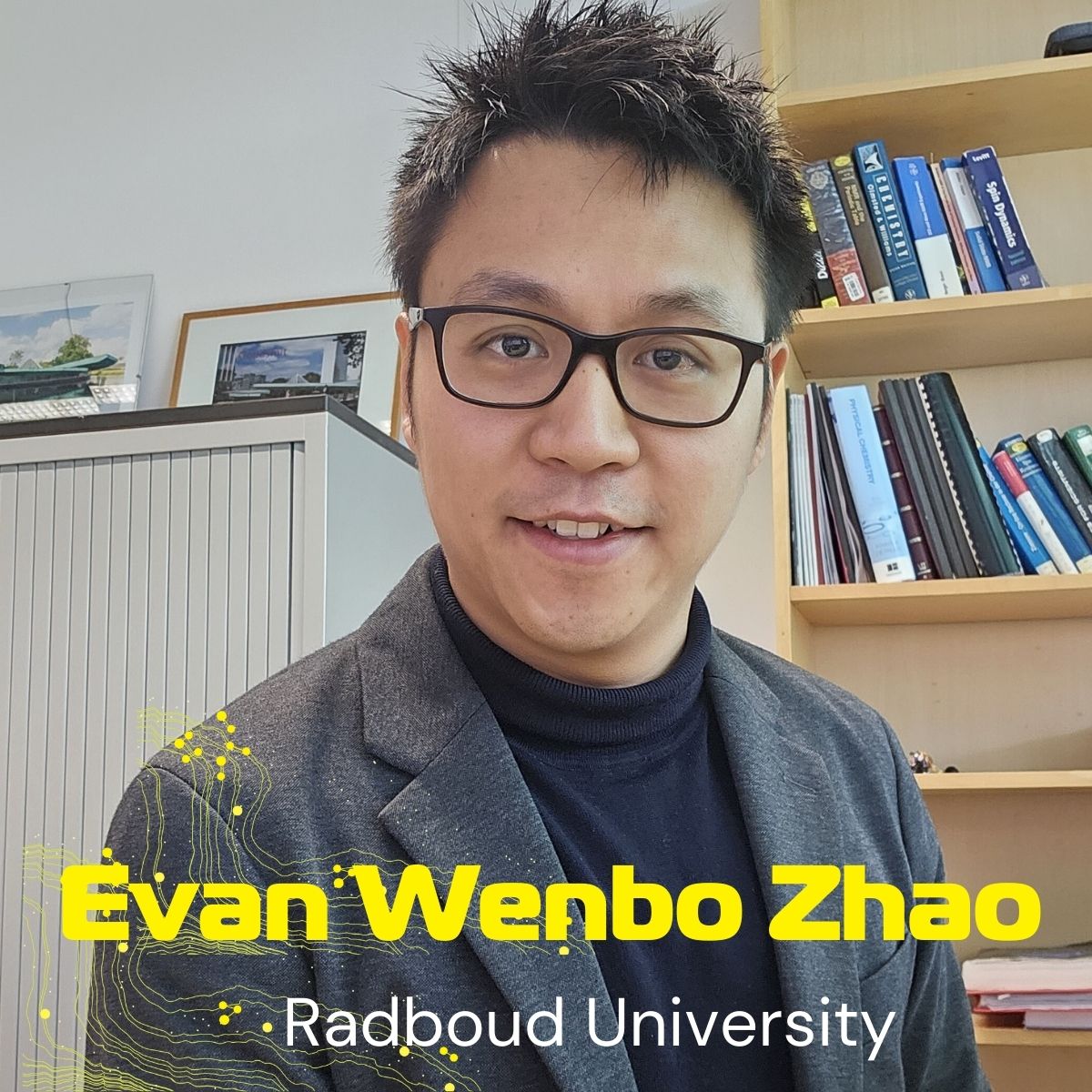
Abstract: Coupling benchtop NMR and EPR for characterizing redox flow batteries
Formation of intermediates and degradation products, ion transport and crossover of active species through membranes are all important processes synergistically affect the performance of a redox flow battery. I will present how we couple nuclear magnetic resonance (NMR) and electron paramagnetic resonance (EPR) to probe these processes in operando. NMR and EPR are non-invasive, atom-specific, quantitative and complementary to one another – the former detecting diamagnetic species and the latter paramagnetic species. Using anthraquinone and viologen-based RFBs as examples, I will demonstrate the detection and quantification of radical intermediates and degradation products. I will show how the electronic structures of the radicals can be revealed through the analysis of the hyperfine coupling features of the EPR spectra. Furthermore, lithium ion transport through the membranes, as a function of charge-discharge cycles, can be captured and quantified, as well as the crossover of the redox-active species. Simultaneously, I will demonstrate that compact and low-cost benchtop NMR and EPR systems have sufficient spectroscopic resolution and sensitivity to perform these mechanistic studies, with the hope of increasing the accessibility to a broader electrochemistry community.
Afternoon break-out sessions
Session 4: Water electrolysis - high temperature
Mihalis Tsampas DIFFER
Dr. Mihalis N. Tsampas received his PhD in catalysis and electrochemistry from the University of Patras in 2010, focusing on electrochemical membrane reactors. Since 2014, he has led the CEPEA group at DIFFER (Netherlands). Previously, he was a postdoc at CNRS (France) on energy storage and conversion. He has 74 papers, 2 book chapters, and 6 patents. His research develops materials and processes for PEM, AEM, SOEC, and PCEC to convert H₂O, CO₂, and N₂ into valuable products, with innovations in electrochemical membrane reactors using light and plasma activation.
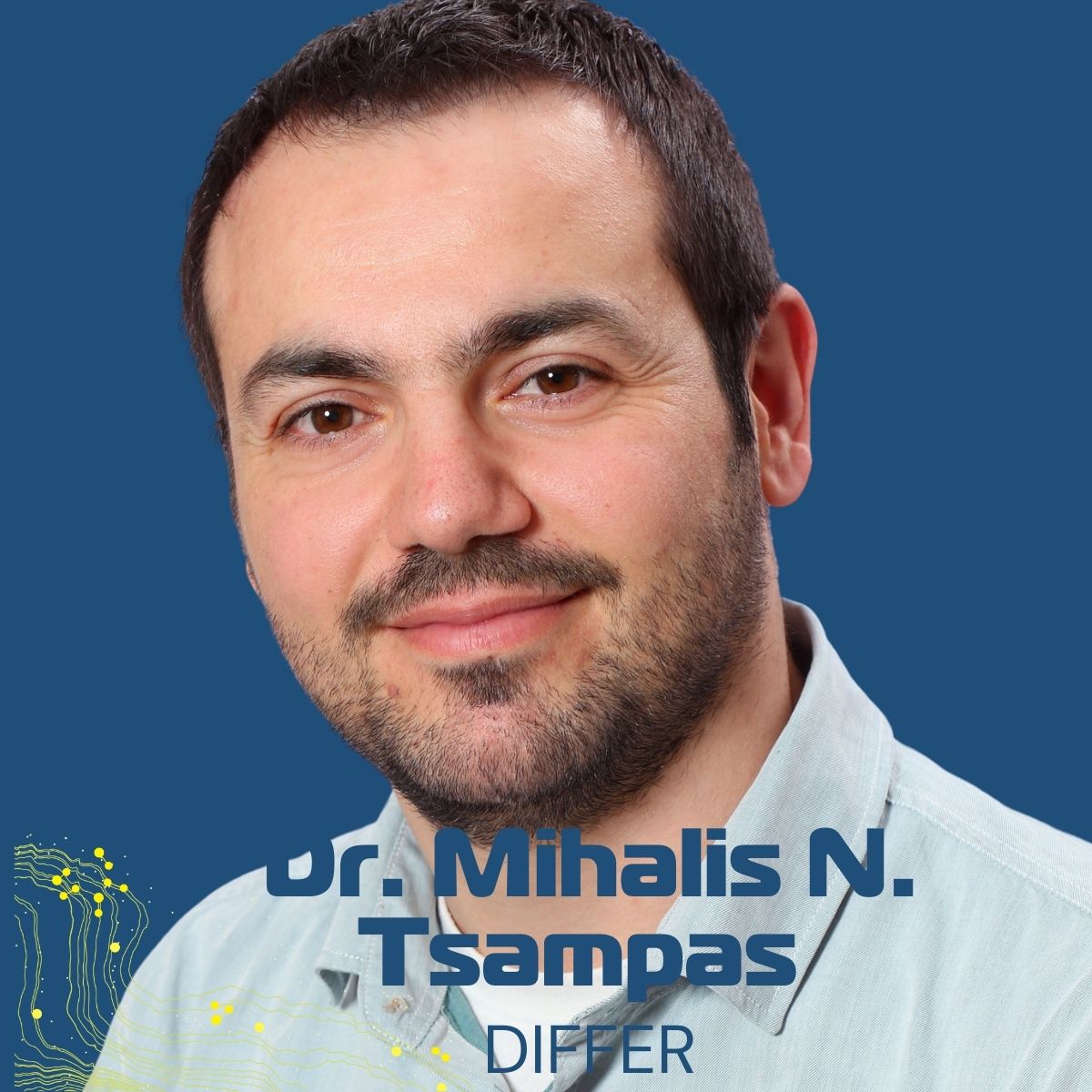
Abstract:
Electrolysis is at the core of Power-to-X (P2X) technologies, enabling the conversion of renewable electricity into fuels and chemicals. Among electrolysis technologies, Solid Oxide Electrolysis Cells (SOECs) and Proton-Conducting Electrolysis Cells (PCECs) stand out for their high efficiency, benefiting from favorable thermodynamics and reaction kinetics at elevated temperatures. A key advantage of these technologies is their reliance on scalable, non-precious materials such as nickel, zirconia, and steel, making them cost-competitive. While SOECs are relatively mature and widely used for the co-electrolysis of CO₂ and H₂O to produce syngas (CO and H₂), PCECs offer a unique advantage by operating at lower temperatures, facilitating the direct production of methane (CH₄), higher hydrocarbons (C₂, C₃), and alcohols. These emerging technologies hold significant potential for enabling carbon-neutral energy cycles and expanding P2X applications.
Despite their promise, SOECs and PCECs face critical degradation challenges that hinder commercialization. SOEC degradation primarily occurs at the stack level, requiring improvements in interconnect coatings, contact materials, and thermal stability. PCECs, still in an earlier stage of development, suffer from single-cell degradation issues, including electrode sintering, proton transport limitations, and chemical instability under CO₂/H₂O electrolysis conditions. In this contribution, we will explore advancements in materials, novel cell architectures, and innovative operation modes—such as coupling with thermal catalysis and plasma activation—aimed at enhancing SOEC and PCEC performance and accelerating their commercial viability.
Session 4: Water electrolysis - high temperature
Vassilis Kyriakou – RUG
Vassilis Kyriakou is an Assistant Professor in Energy Conversion and Processes at RUG. He holds a PhD and a Diploma in Chemical Engineering, both from the Aristotle University of Thessaloniki and he has been as postdoctoral researcher in Yildiz Lab at MIT, the Dutch Institute for Fundamental Energy Research (DIFFER), and CERTH. Vassilis’ research focuses on electrochemical reaction engineering, catalysis and materials for solid oxide, protonic ceramic and AEM cells towards the production of chemicals, fuels and/or power from sustainable feedstocks. He is currently involved in several important projects on high temperature electrolysers and fuel cells, such as HyUSE and HyPRO of GroenvermogenNL.
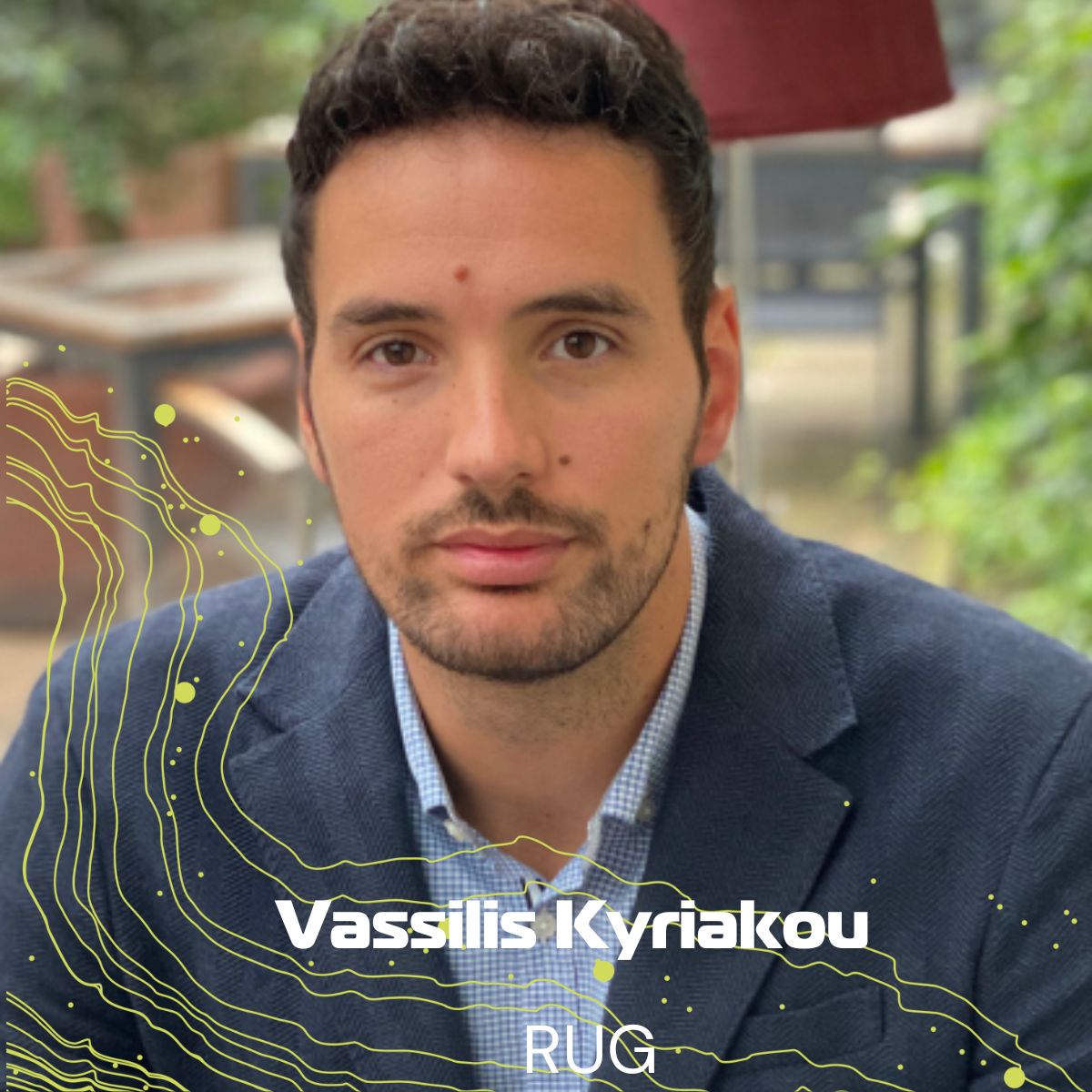
Nanoengineered Mixed Ionic-Electronic Conducting Electrocatalysts for Green Hydrogen Production and Storage at Industrially Relevant Temperatures
Chemical energy storage is the transformation of electrical power into chemical energy carriers, allowing grid flexibility by backing up intermittent electricity of renewable energy sources. Once the excess renewable energy is stored into chemical bonds, several pathways are opened for both re-electrification and mobility. For such storage, efficient technologies for electrosynthesis of chemicals and fuels are critical for a carbon-free energy future. Here, I will discuss about the generation and simultaneous conversion of green hydrogen in oxide ion- and proton- conducting ceramic cells, operating at intermediate and high temperatures. I will focus on rationally designed, nanoengineered mixed ionic-electronic conducting electrocatalysts, prepared by redox exsolution that allow promising performance and stability in harsh environments. The proposed approaches aim to bridge electrochemistry and heterogeneous catalysis, while exemplifying a route for enabling electrosynthesis at industrially relevant temperatures.
Session 5: (Bio) CO2 electrochemical conversions 2
Amanda Garcia – UvA
Dr. Amanda Garcia is an Assistant Professor at the University of Amsterdam’s Van’t Hoff Institute for Molecular Sciences. She obtained her Ph.D. in Physical Chemistry from São Paulo University/Brazil. Before her tenure at the University of Amsterdam, Amanda was an Electrochemistry Scientist at TNO-Delft, the Dutch Institute for Applied Science, and held postdoc positions at Leiden University/NL and Université de Poitiers/France. Dr. Garcia is also involved with the Electrochemical Conversion & Materials (ECCM) community in NL and has led several ECCM-funded projects on carbon capture and utilization, aimed at overcoming technological challenges and enhancing the acceptance of new technologies. Her research focuses on electrocatalysis and electrocatalytic synthesis using renewable resources to create valuable chemical products and understanding these transformations at the mechanistic and atomic level.
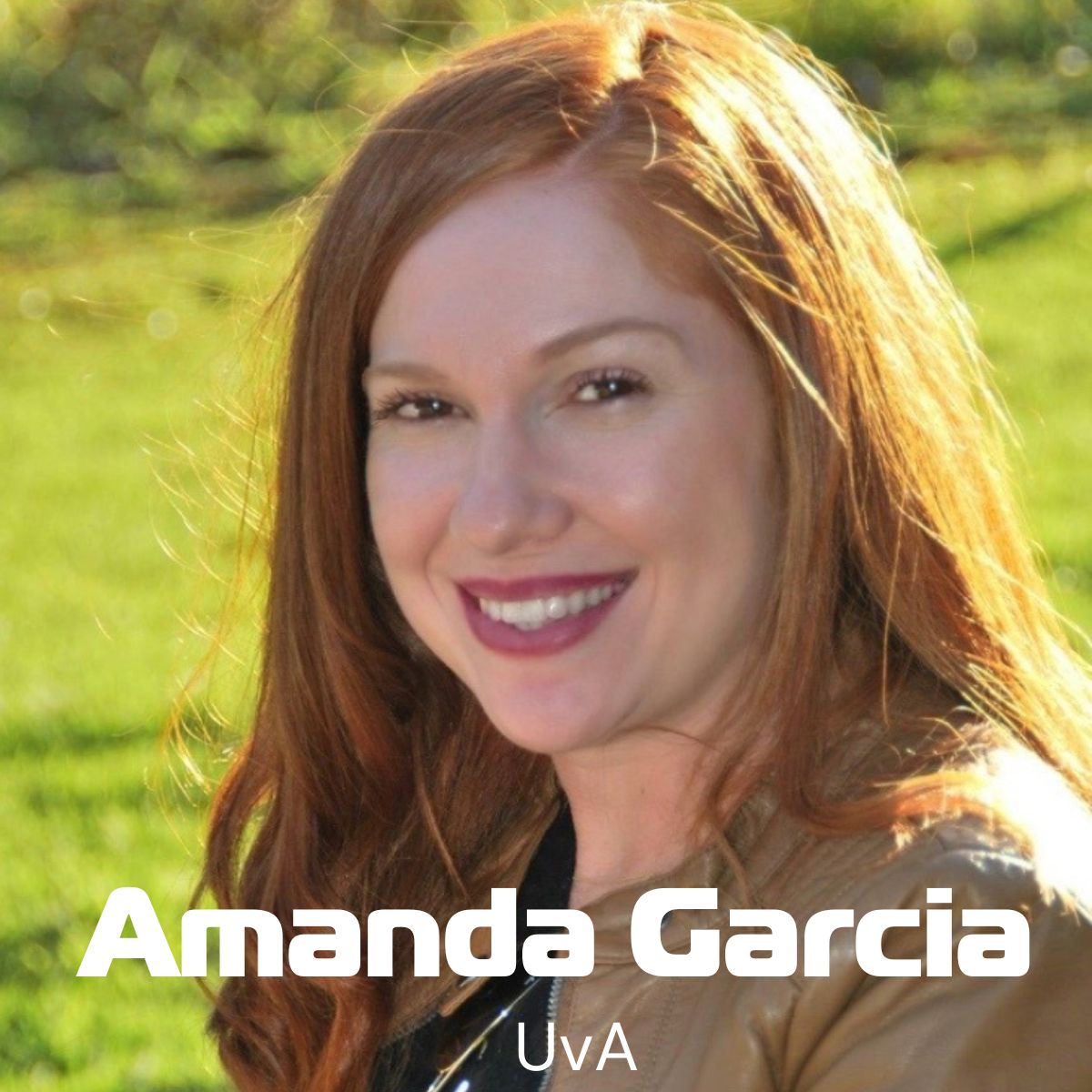
Abstract: Exploring the Electrochemical Reduction of CO2 on Copper Electrodes in Organic Solvents
This lecture delves into the fundamental principles underlying the electrochemical reduction of carbon dioxide (CO2RR) and its interaction with the hydrogen evolution reaction (HER). Our focus is on our group’s extensive exploration of CO2RR on copper electrodes in organic solvents.
Utilizing online gas chromatography and in situ FTIR spectroscopy, our findings underscore the significant impact of electrode morphology and solvent composition on this reaction. Notably, in solvents such as acetonitrile, our analyses reveal the critical role of water content at the solid-liquid interface in determining reaction selectivity. We also demonstrate that the CO2 reduction reaction competes with HER under these conditions.
Building on prior observations in aqueous solvents, our study elucidates the decisive influence of alkyl cation length—commonly used as an electrolyte in organic solvents—on the reaction mechanism. Specifically, our data show that smaller cations (TEA) promote the formation of oxalic acid, while larger cations (TBA) favor the production of carbon monoxide.
Moreover, we investigated the impact of amine solvents on carbon capture and utilization in a direct process. Our in situ spectroscopic analysis indicates that the steric effects of amines and their interactions with the electrode surface significantly influence product distribution during CO2RR.
This lecture will leverage advanced in situ spectroscopic techniques and DFT calculations to elucidate the complex mechanisms behind these reactions and highlight the pivotal role of the cation in determining product distribution.
Session 5: (Bio) CO2 electrochemical conversions 2
Arnaud Thevenon – UU
Arnaud Thevenon received his PhD (2018) from the University of Oxford. He was a Marie Skłodowska-Curie postdoctoral researcher at Caltech (2018–2021) and the University of Konstanz (2021) before joining Utrecht University as an Assistant Professor in 2021. His research interest includes the development of homogeneous thermo/electrocatalysts for small molecules, biomass, and waste (plastic) valorization as well as the development of novel polymers that are intrinsically circular by design.
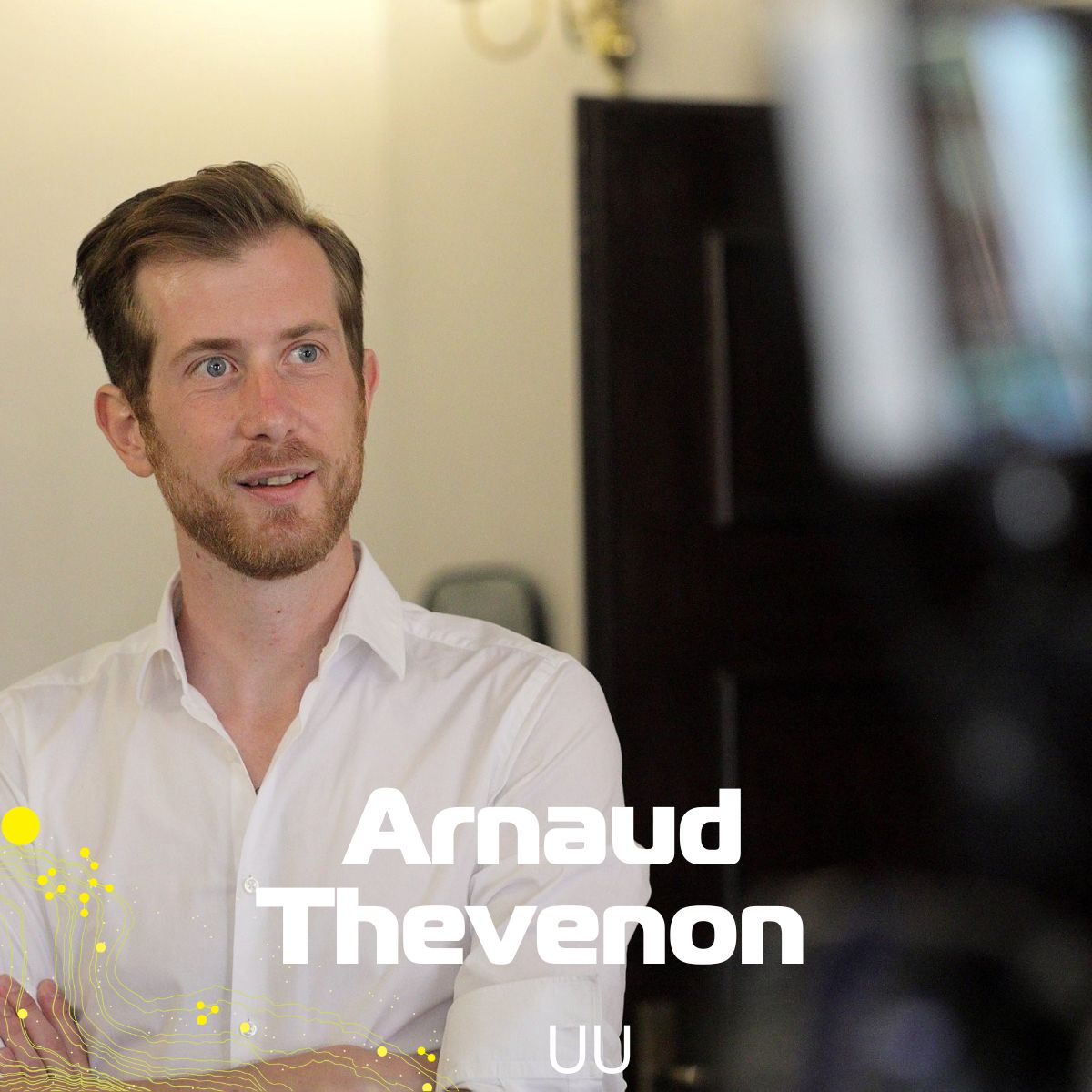
Abstract
The electrocatalytic conversion of CO₂ to hydrocarbons using renewable energy offers a sustainable pathway for producing carbon-neutral fuels and value-added chemicals.1 However, a major challenge remains in developing efficient and robust electrocatalysts with high selectivity for a single product at low overpotential and long stability.1 Here, we report the use of molecular additives electrodeposited on heterogeneous electrodes to fine-tune the microenvironment of active sites. These hybrid electrodes exhibit superior selectivity, activity, and stability compared to their pristine counterparts under similar conditions.2 We identified multiple roles of the organic coating, including nanostructuring and stabilizing metallic electrodes during catalysis, stabilizing key intermediates, forming hydrophobic layers, and/or serving as active catalytic sites.3-5 We demonstrate that this approach is versatile across various systems and holds significant promise for advancing next generation electrolyzers.
(1) Z. Sun et al. Chem 2017, 3, 560–587.
(2) D.-H. Nam et al. Nat. Mater., 2020, 19, 266–276.
(3) A. Thevenon et al. Chem. Int. Ed. 2019, 18, 58 (47) 16952
(4) F. Li et al. Nature, 2020, 577, 509-513
(5) A. Thevenon et al. ACS Catal. 2021, 11, 8, 4530–4537
Session 6: Electrochemical production of NH3, C-N and specialty chemicals
Associate Prof Alexander Bagger, Technical University of Denmark (DTU)
Alexander Bagger is an associate professor at the Department of Physics, at the Technical University of Denmark. He received his PhD (2019) in Theoretical Electrochemistry at University of copenhagen with Prof. Jan Rossmeisl. Following his PhD, he had a short exit into industry at IBM. Back in academia he won the Carlsberg Foundation Internationalisation Fellowships grant for a research stay at Imperial College London with Prof. Ifan Stephens. And recently he has been building his group at DTU useing atomistic simulations to understand selectivity challenges at the atomic scale for electrochemistry reactions.
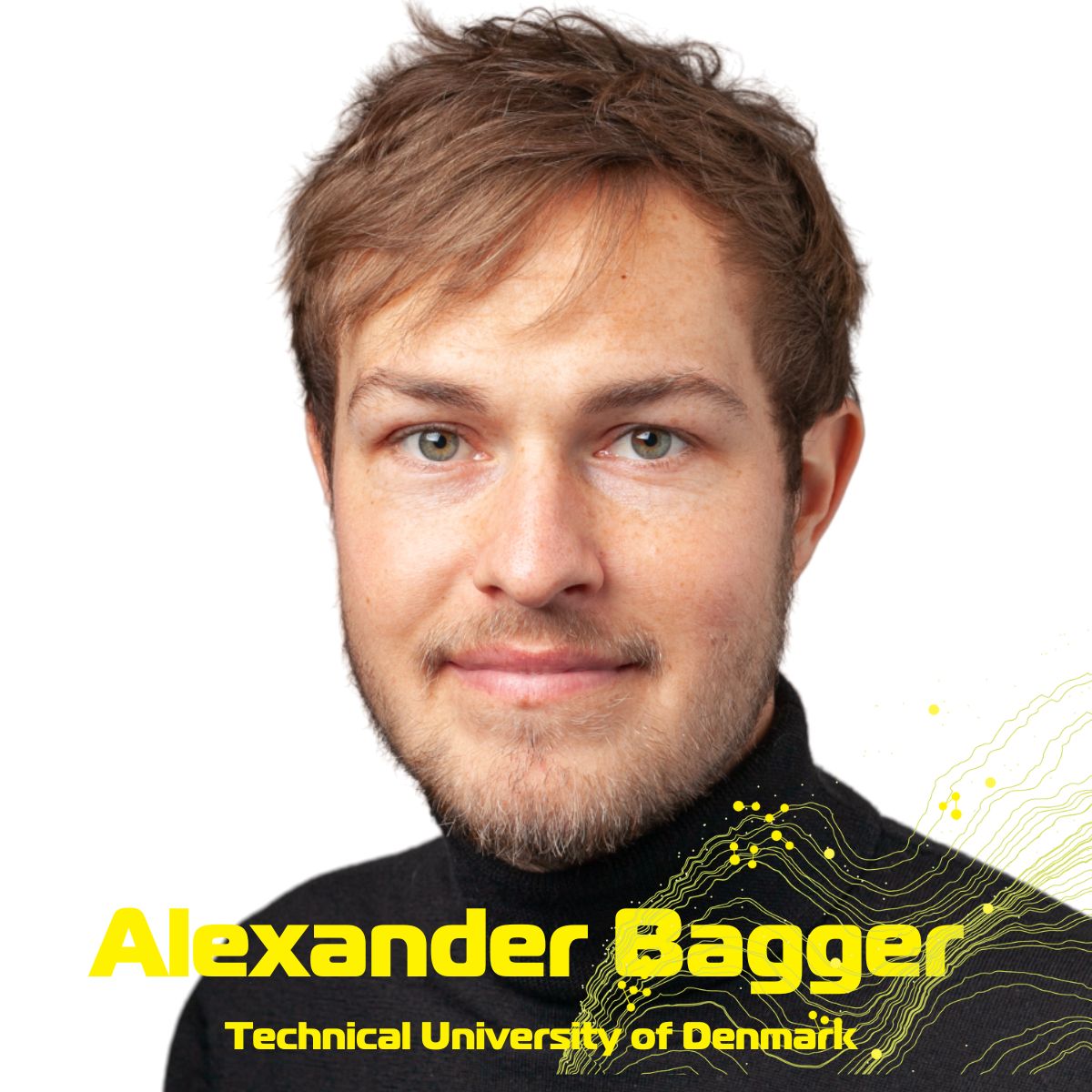
Abstract: Computations for Electrochemical Production of NH3, C-N and Specialty Chemicals
Alexander Bagger Technical University of Denmark, Department of Physics
I will discuss how experiments and computational simulations can support each other. I will focus on electrochemical reduction of NOx, CO2, N2, and the combinations. Importantly, all these reactions share a direct competition with hydrogen, and furthermore, several products are formed from each reactant of these reactants. I will give minimalistic models that do not overfit or over interpretating experimental data:
- eCO2 reduction show multiple different products depending on metal catalyst1,2
- eNOx reduction produce N2O, N2 and NH33,4. Interestingly, reactive metals work close to their reduction potential5-6.
- eN2 reduction to NH3 at ambient conditions has been confirmed on a Li-mediate system7. I will discuss systems beyond lithium8.
- Finally, I will show predictive schemes for the synthesis of urea9,10.
[1] Hori et. al., J. Chem. Soc., Faraday Trans., 1989.
[2] Bagger et. al., ChemPhysChem, 2017.
[3] Rosca, Duca, Groot, Koper, Chem. Rev. 2009.
[4] Wan, Bagger, Rossmeisl, Angew. Chem., 2021.
[5] Carvalho, …, Stoerzinger, JACS 2022.
[6] Riyaz, Bagger, Electrochim. Acta. 2025.
[7] Tsuneto et al. JEAC. 1994.
[8] Bagger, et. al., ACS Energy Letters. 2024.
[9] Wan, …, Bagger, ACS Catalysis, 2023.
[10] Wuttke, Bagger, Commun. Chem. 2025.
Session 6: Electrochemical production of NH3, C-N and specialty chemicals
Dulce Morales – RUG
Dulce M. Morales has a background in Industrial Chemical Engineering from Instituto Politécnico Nacional, México (2012), and received her Master (2015) and Dr. rer. nat. (2019) degrees from Ruhr-Universität Bochum in Germany. She is presently an assistant professor in the Engineering and Technology Institute Groningen at University of Groningen in the Netherlands. Her research focuses on the electrochemical conversion of renewable resources to fuels and value-added chemicals.
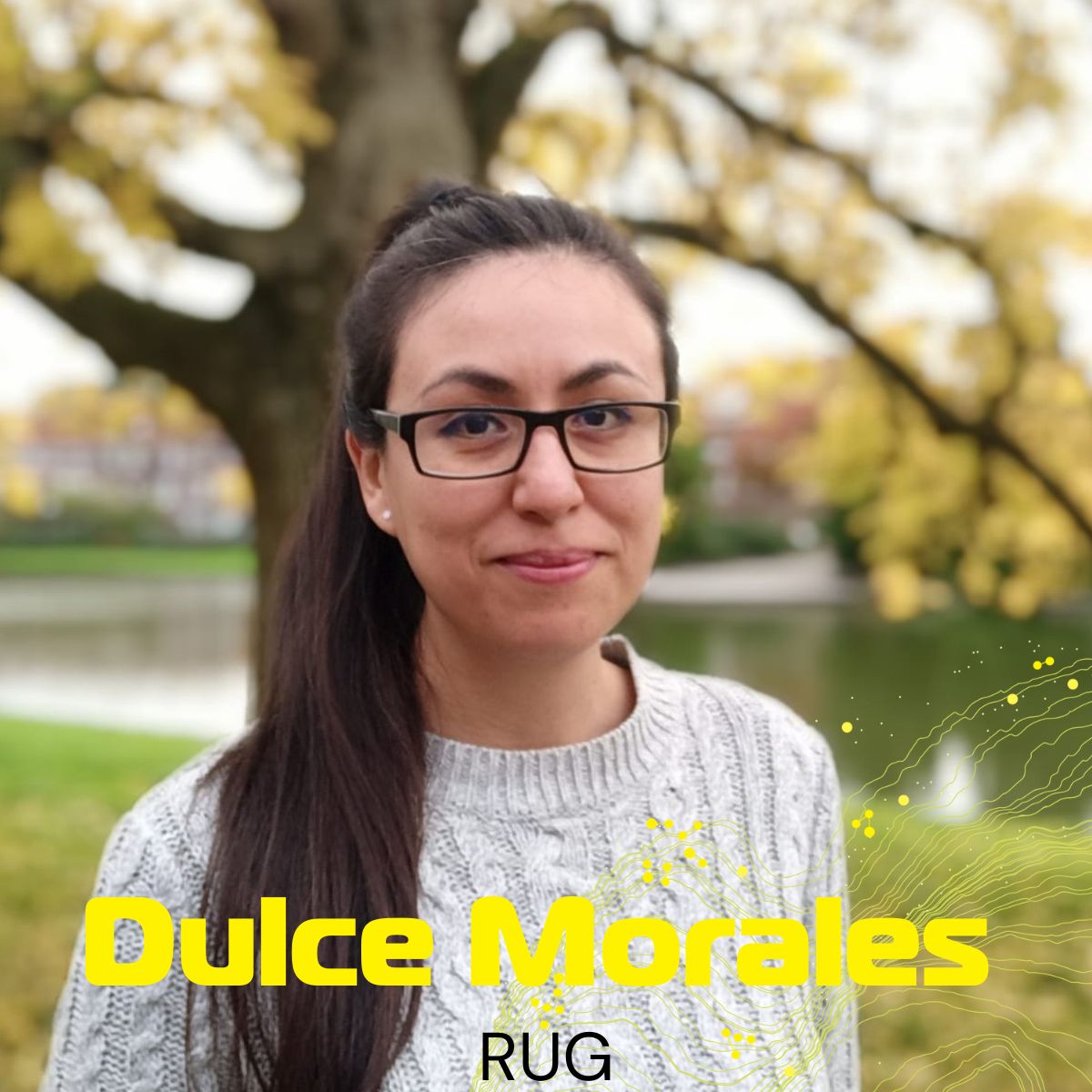
Abstract: Electrooxidation of alcohols in hybrid water electrolysis: activity vs selectivity
Integrating the electrooxidation of an organic compound, such as an alcohol, in a water electrolyzer is a promising strategy for saving energy during hydrogen production while forming value-added products at the anode. This approach is known as hybrid water electrolysis (HWE). In this contribution, the concept of “energy saving” in HWE is illustrated by comparing the performance of a series of LaFe1xCoxO3 perovskites towards the oxygen evolution, and the electrooxidation of glycerol and isopropanol.[1] As a second example, the influence of various operating conditions, including electrode potential and electrolyte composition, on the performance of Ni oxide nanoparticles towards the electrooxidation of glycerol is discussed.[2] The results shown here illustrate the trade-off between activity and selectivity in HWE, and highlight the need for identifying suitable catalysts and electrolysis conditions to minimize this trade-off.[3]
References: [1] A.C. Brix, et al. ChemElectroChem 9 (2021) e202200092 [2] D.M. Morales, et al. ACS Catalysis 12 (2022) 982-992 [3] F.J.A. van Lieshout & D.M. Morales, ChemPlusChem 89 (2024) e202400182.
Plenary panel | Dr Andrea Ramírez Ramírez
Dr Andrea Ramírez Ramírez is professor in Low Carbon Systems and Technologies at TU Delft. She holds a BSc in chemical engineering, a MSc in Human Ecology, and a PhD in industrial energy efficiency. Her research focuses on the ex-ante evaluation of low-carbon technologies and the design of methodologies and tools to assess their potential contribution to sustainable industrial systems. Prof Ramírez is a fellow of the Netherlands Academy of Engineering and head of the department of Chemical Engineering at TU Delft.
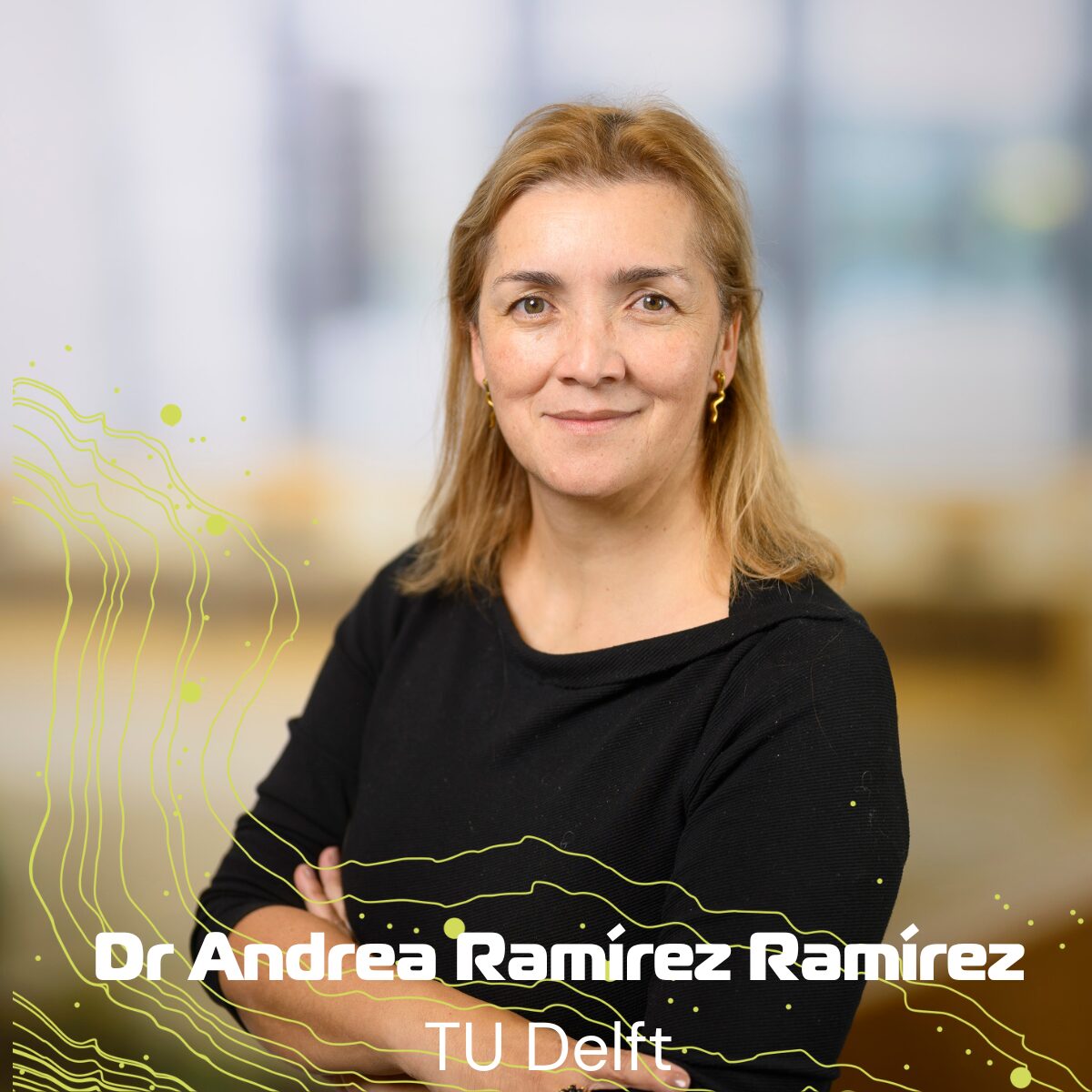
Plenary panel | Earl Goetheer
Earl Goetheer has more than 25 years of experience as a chemical engineer. He is now working as a CTO at HighTechXL (a venture building company). He was the principal scientist Process Technology at TNO and a professor at the TUDelft. He has published more than 100 articles and has more than 70 patent (applications) in the field of (electro) chemical engineering.
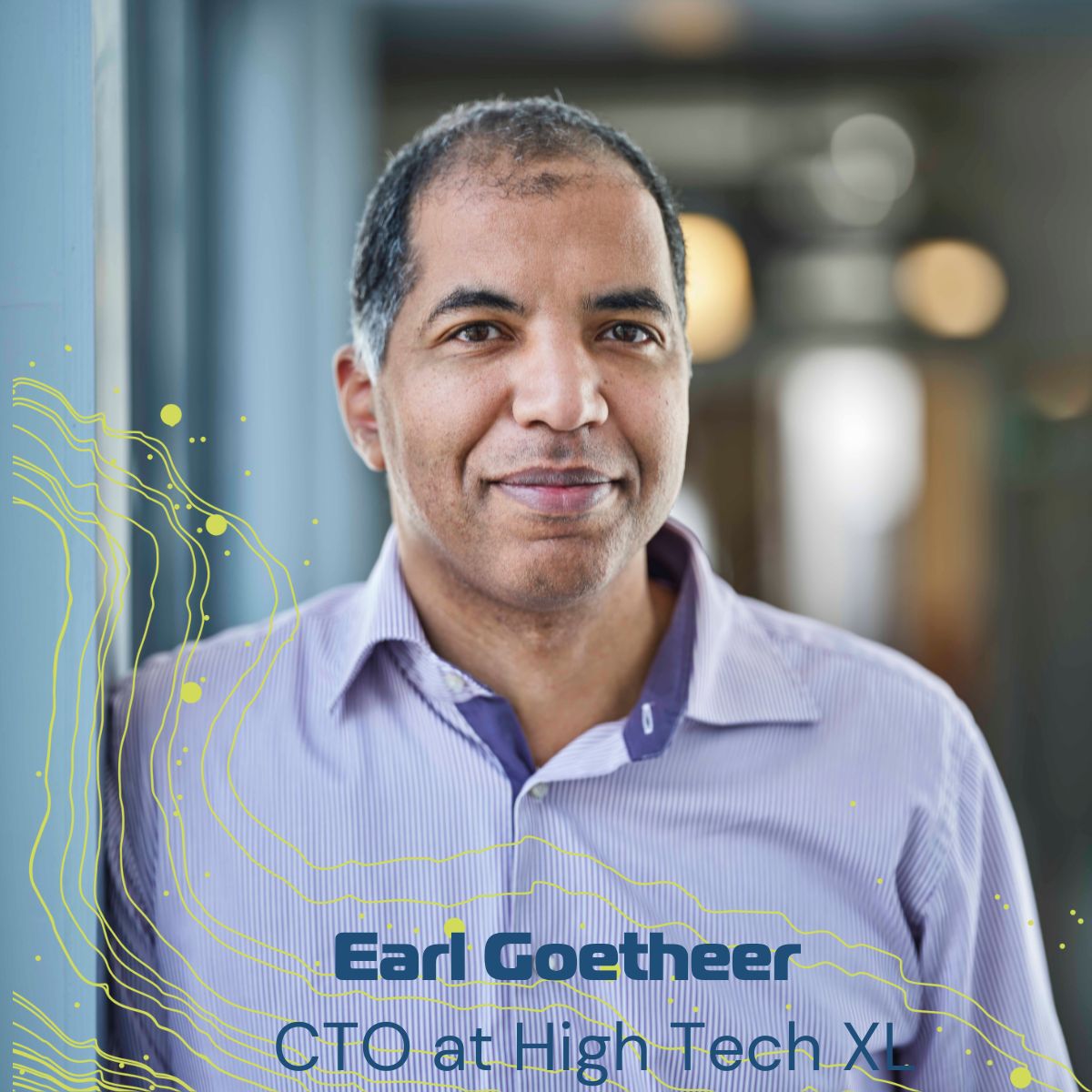
Plenary panel | Moniek Tromp
Moniek Tromp obtained her MSc and PhD at the University of Utrecht. Moniek held positions in the UK and Germany, and from July 2018 she has taken up the Chair of Materials Chemistry at the Zernike Institute at the University of Groningen, where, from January 2023, she is also the research director of the Zernike Institute for Advanced Materials and co-Director of the Groningen Engineering Center. Moniek was chair and figure head of the board acquiring a National Growth Fund Project (‘Materials Independence and Sustainable Batteries’) and is in the steering board of the Battery Competence Cluster NL. She currently is the Captain of Science of the Topsector Chemistry.
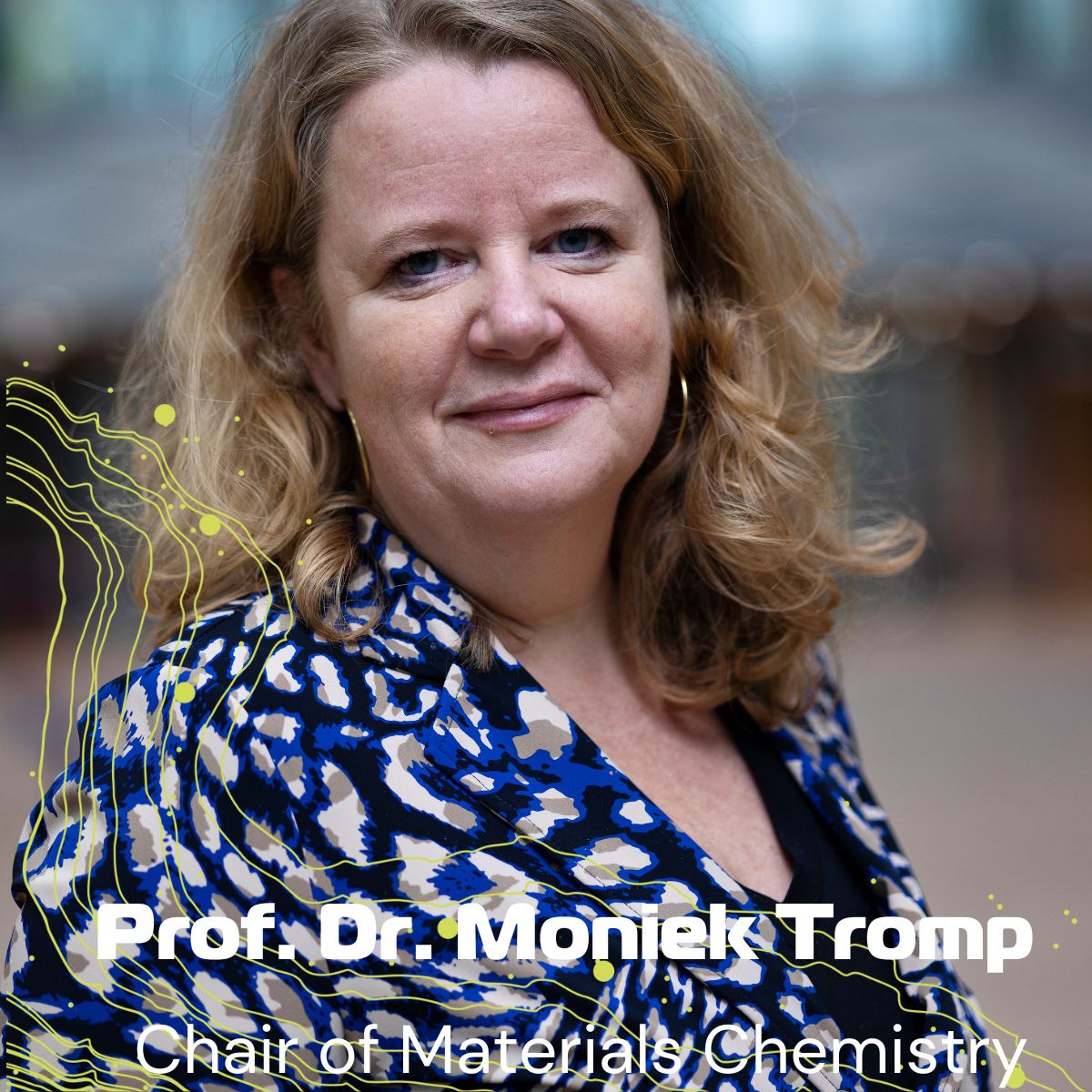
Plenary panel | Martijn de Graaff
Martijn is responsible for strategic business management for TNO’s programs in the field of Industrial Transformation, Power-2-X, Hydrogen and CCU. He works at the intersection of multiple technologies, products, companies and market segments to accelerate the transition to a CO2-neutral and circular economy. Previously, he worked in various business development positions focused on innovation in the chemical, process technology and high-tech industries. He is deeply involved in setting up public-private partnerships at regional, national and transnational levels, with a focus on industrial transformation and electrification in Northwestern Europe.
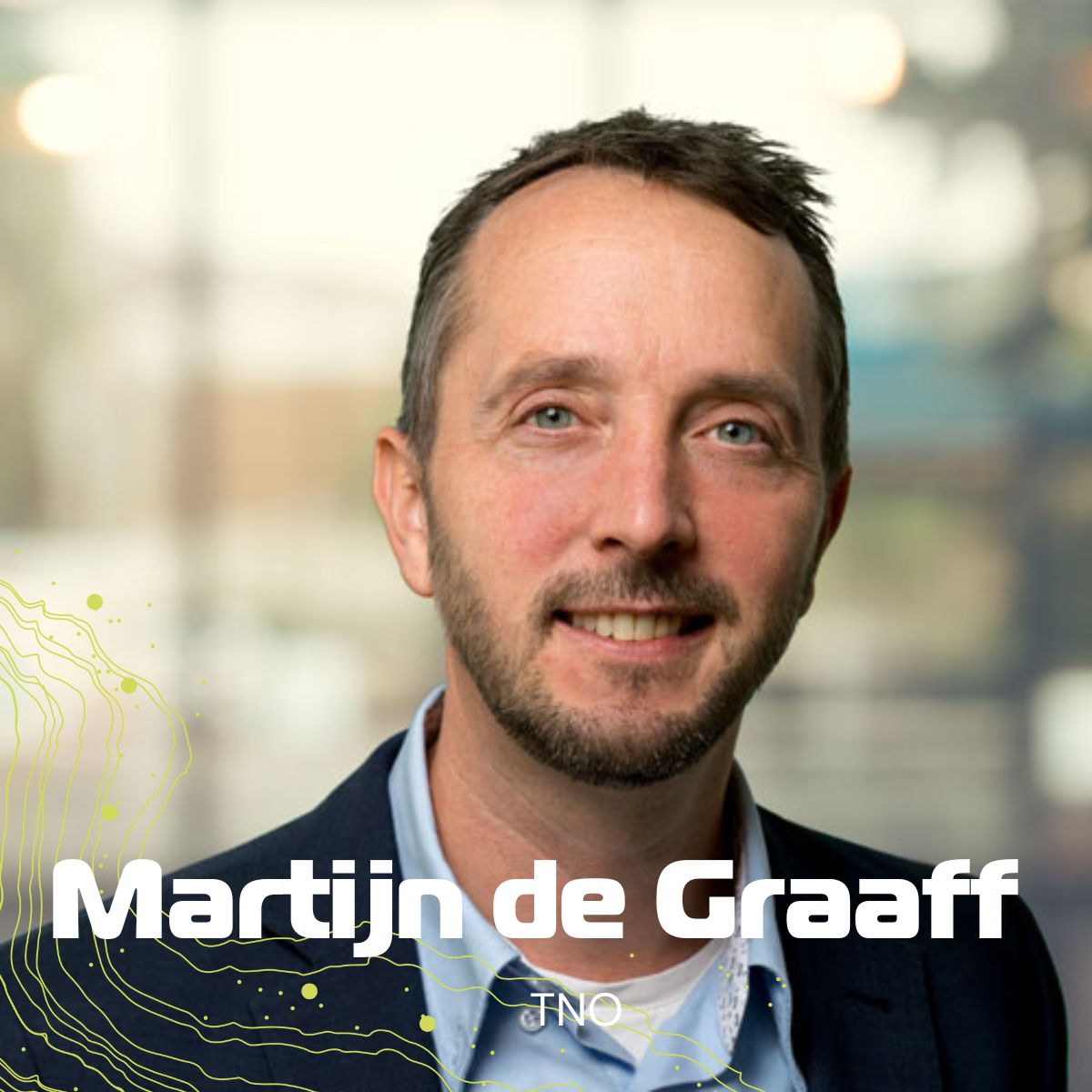